contributed by
Dr. Robert E. LaPointe1
Novomer
General Information
The transition metal catalyzed polymerizaion of olefins can be thought of as a reaction in which the carbon-carbon π-bond in α-olefins (1-alkenes) are cut and then remade as a carbon-carbon σ-bonds, linking the olefins together in long hydrocarbon chains. This reaction is exothermic by ~ 20 Kcal/mol, the energy difference between the carbon-carbon
π- and σ-bonds. For typical early transition metal, d0 catalysts, the activation energy is ~ 10 Kcal/mole, making this a very facile reaction.

These long chain macromolecules are known as polymers (from the Greek,
poly = many and mer = units) and are the principal constituents of many common
plastics. Some familar plastics made via early transition metal catalyzed polymerization include: high density polyethylene (HDPE, R=H), linear low density polyethylene (LLDPE, R = mostly H with some Et, Bu or Hx), polypropylene (R = Me) and ethylene-propylene-diene-modified rubber (EPDM, R = H, Me and Alkenyl).
Mechanism
The commonly accepted mechanism for the olefin polymerization reaction is shown below. A severely electron deficient metal center coordinates the π-bond of an olefin to form a weakly bound olefin complex; the bond is weak because there are no d-electrons to form a π-bond. The bound olefin then inserts into a metal-carbon bond within the complex via a four center transition state, forming new metal-carbon and carbon-carbon
bonds. This process then repeats, extending the chain indefinitely.

Chain growth can be terminated by a number of processes. One of the most impotant is β-hydride elimination. Since this is simply the reverse of insertion, the activation energy is ~ 30 Kcal/mole plus the energy difference between the metal-carbon and metal-hydrogen bonds. Catalysts with relatively strong M-C bonds give higher molecular weight polymers, while those with relatively strong M-H bonds give lower molecular weight polymers (waxes). Beta-hydride elimination leads to polymers with vinyl (R=H) or vinylidene (R=Me, Et, Pr...) end groups. The vinyl terminated polymers are, of course, α-olefins and may also be incorporated into the growing polymer chains giving "long chain branched" products under appropriate conditions.
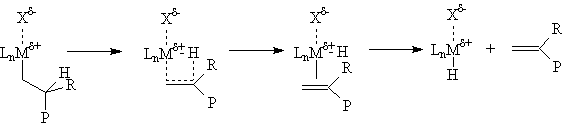
Another termination process of great practical use is hydrogenolysis. This sigma-bond metathesis reaction leads to polymers with saturated end
groups:
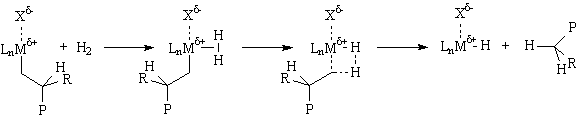
Catalysts
There have been many generations of olefin polymerization catalysts:
-
"Black Box" heterogeneous catalysts consisting of a high valent transition metal halide, oxide or oxo-halide with an alkylating co-catalyst such as an alkyl aluminum were first described by Karl Ziegler and Guilio Natta. Most of these catalyst systems are prepared on supports. Classic examples, many of which are still used today, include TiCl4/MgCl2/AlEt3 (LLDPE, Dow), CrO3/Al2O3/AlEt3 (HDPE, Phillips) and VOCl3/AlEt3 (EPDM, Shell).
While these catalysts are exceedingly active, they have an exceedingly
low tolerance for functional groups because of their Lewis acidic nature.
Typically they contain multiple active sites with varying, but generally
poor, reactivity ratios for α-olefins relative
to ethylene. Little is known about the nature of the actual catalytic
species in these systems.
-
"Black Box" homogeneous catalysts consisting of the high valent transition
metal complex Cp2ZrCl2 in combination with methylalumoxane ([MeAlO]n, MAO, a hydrolysis product of AlMe3) was first described by Walter Kaminsky (Sinn, H.; Kaminsky, W. Adv. Organomet. Chem. 1980, 18, 99) and was active for the homopolymerization of ethylene to high density polyethylene.
This class of catalysts came to include rationally designed systems, such as Ewen's Et(Ind)2ZrCl2/MAO (isotactic polypropylene: Ewen, J. A., J. Am. Chem Soc. 1984, 106, 6355-6364), and iPr(Cp)(Flr)ZrCl2/MAO (syndiotactic polypropylene: Ewen, J. A.; Jones, R. L.; Razavi, A.; Ferrara, J. D., J. Am. Chem. Soc. 1988, 110, 6255-6256). These catalysts rejuvenated olefin polymerization catalysis research in the early 1980's. Despite a quarter century of intense
academic and industrial research, the nature of MAO is still largly unknown.
Active site counting studies and chromatographic analysis suggest that the MAO exists as clusters with ~10-15 aluminum atoms and acts as both a methyl transfer agent and a Lewis acid to form anions which are weakly coordinating to the cationic metal methyl complexes which are the active
catalysts.
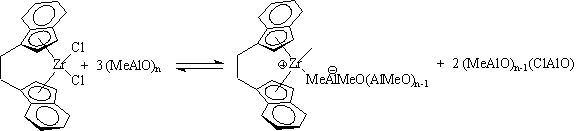
These catalysts have good activity and remarkable tunability via ligand
changes in the pre-catalyst complex. For example, the chiral active
site of the Kaminsky-Brintzinger catalyst system (Kaminsky, W.; Kulper,
K.; Brintzinger, H.H.; Wild, F.R.W.P. Angew. Chem. Int. Ed. Eng.1985,
24,
507.) depicted above generates isotactic polypropylene. However,
the use of large amounts of MAO (~ 1000 equivalents, presumably to drive
the
above equilibrium to the right) results in high catalyst cost and catalyst
residue loading of the polymer.
-
Cationic homogeneous catalysts with weakly coordinating anions were first
described by Richard Jordan (Jordan, R.F.; LaPointe, R.E.; Bajgur, C.S.;
Echols, S.F.; Willett, R. J. Am. Chem. Soc. 1987, 109,
4111.) and prepared via the reaction of early transition metal alkyl complexes,
such as Cp'2ZrMe2 with oxidizing tetraphenylborate
salts including AgBPh4 and (Cp2Fe)BPh4.
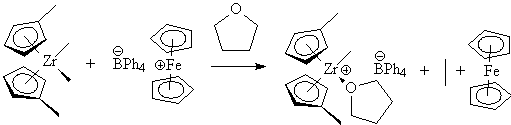
Like the Kaminsky type catalysts, the Jordan type catalysts have exceptional
tunability via the metal ligands. The early versions of these catalysts
showed only modest activity, due to competition for the active site between
the olefin and the ether.
-
After the identification of the active site as an alkyl metal cation, rapid
advances in pre-catalyst ligand design and activator systems were made
by both industrial and academic researchers. New ligands produced
catalyst systems with improved comonomer incorporation (1 and 2,
Dow, for ethylene/1-octene and ethylene styrene, respectively), tacticity,
molecular weight, and even variable tacticity (3, Waymouth, rotation
of the ligands switches the active site symmetry allowing production of
block atactic/isotactic polypropylene elastomer). New anions (4,
Exxon; 5 Marks; 6, Dow) and activators give catalyst systems
with astonishing activities (approaching 50,000,000 grams of polymer/gram
of metal) in industrial reactors.
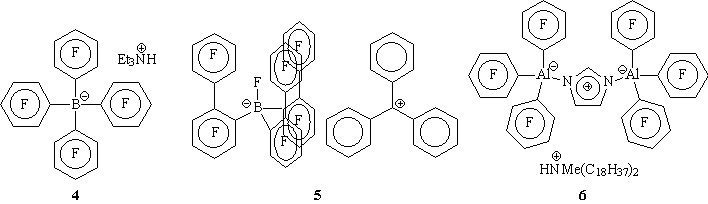
Further Reading and References
[Index] [Keyword Search] [Books & Software]
[ILPI Home Page]
Please visit our sponsor to thank them for supporting this site!
This page was last updated Tuesday, March 31, 2015
This document and associated figures are copyright 1996-2024 by Rob Toreki or the contributing author (if any) noted above. Send comments, kudos and suggestions to us by email. All rights reserved.