General Information
Carbonyl Complexes are compounds that contain carbon monoxide as a coordinated ligand. Carbon monoxide is a common ligand in transition metal chemistry, in part due to the synergistic nature of its bonding to transition metals. We can describe the bonding of CO to a metal as consisting of two components. The first component is a two electron donation of the lone pair on carbon (coordination exclusively through the oxygen is extremely rare) into a vacant metal d-orbital. This electron donation makes the metal more electron rich, and in order to compensate for this increased electron density, a filled metal d-orbital may interact with the empty pi* orbital on the carbonyl ligand to relieve itself of the added electron density. This second component is called pi-backbonding or pi-backdonation. This is shown diagramatically as well as through a simple MO picture below. The MO has been color coded so you can identify each component of the bonding interaction: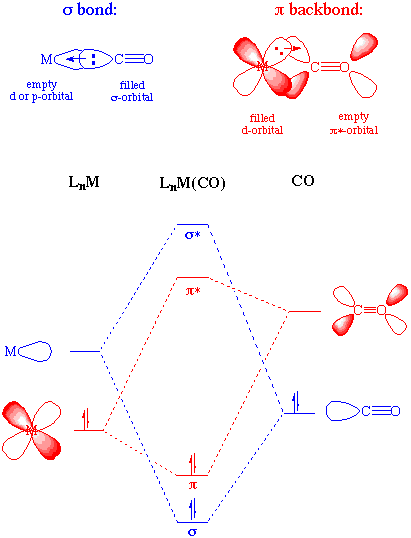
The two components of this bonding are synergistic. The more sigma donation by the carbonyl (or other sigma-donors on the metal center), the stronger the pi-backbonding interaction. Notice that although this involves the occupation of a pi* orbital on the CO, it is still a bonding interaction as far as the metal center is concerned. There is a fundamental similarity between the nature of carbonyl-metal bonding and that of alkenes, acetylenes, phosphines, and dihydrogen.
This occupation of the pi* on CO does lead to a decreased bond order in the carbon monoxide molecule itself. As we might expect, as the pi-backdonation becomes stronger, the CO bond order should decrease from that of the free ligand. Two consequences that we might expect if the CO bond order was reduced would be a lengthening of the C-O bond and a decrease in the carbonyl stretching frequency in the IR. Both of these hold true.
Bonding modes of CO
CO typically bonds in an end-on fashion through carbon as shown above. However, bridging carbonyls are not uncommon and often undergo exchange with terminal carbonyls. A variant of a bridging carbonyl is the "semi-bridging" carbonyl in which the M-CO-M bond is asymmetric rather than symmetric. Some less common bonding modes are shown below; notice that the metrical data provided in each case are consistent with a decrease in the CO bond order: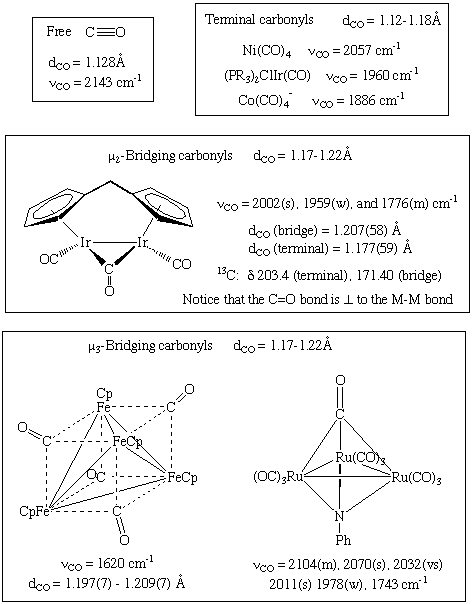
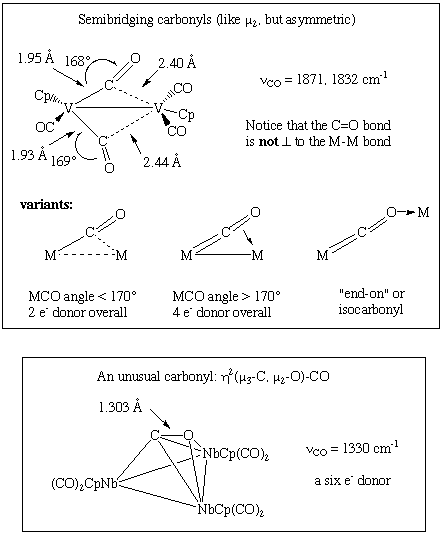
Spectroscopic Features of Carbonyl Complexes
In the IR, typical stretching frequencies are:
- Uncoordinated or "free" CO: 2143 cm-1
- Terminal M-CO: 2125 to 1850 cm-1
- Doubly bridging (mu-2): 1850 to 1750 cm-1
- Triply bridging (mu-3): 1675 to 1600 cm-1
- Semibridging: somewhere between terminal and mu-2.
Two convenient trends are observed in the IR spectra of carbonyl complexes that are both consistent with the concept of pi-backbonding discussed above:
- With each charge added to the metal center, the CO stretching frequency decreases by approximately 100 cm-1.
- The better the sigma-donating capability (or worse the pi-acceptor ability) of the other ligands on the metal, the lower the CO stretching frequency.
For simple carbonyl complexes, counting the number of IR and Raman CO stretching frequencies will often permit one to make a structural assignment. The number of CO stretches expected for possible geometries/isomers can be predicted using group theory and the calculated results compared to the experimental data.
In the 13C NMR spectrum, coordinated carbonyl ligands typically appear in the range of 180 to 250 ppm. Isotopically enriched carbonyl complexes are often prepared to simplify mechanistic investigations or to facilitate the collection of the spectrum. In addition, the coupling of a 13C-enriched complex to other spin active nuclei such as 103Rh or 31P, can aid in structural assignments.
Syntheses of metal carbonyls
Metal carbonyls can be made in a variety of ways. Here are just a few examples:
For Ni and Fe, the homoleptic or binary metal carbonyls can be made by the direct interaction with the metal (Equation 1).
In other cases, a reduction of a metal precursor in the presence of CO (or using CO as the reductant) is used (Equations 2-3).
Carbon monoxide also reacts with various metal complexes, most typically filling a vacant coordination site (Equation 4) or performing a ligand substitution reactions (Equation 5).
Occasionally, CO ligands are derived from the reaction of a coordinated ligand through a deinsertion reaction (Equation 6).
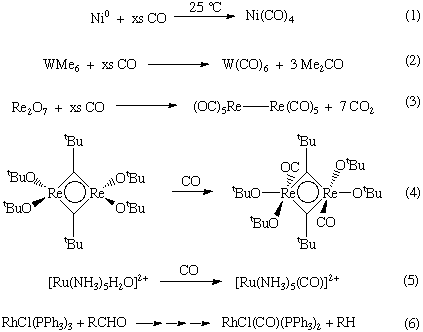
[Index] [Keyword Search] [Books & Software]
[ILPI Home Page]
Please visit our sponsor to thank them for supporting this site!
This page was last updated Tuesday, March 31, 2015
This document and associated figures are copyright 1996-2024 by Rob Toreki or the contributing author (if any) noted above. Send comments, kudos and suggestions to us by email. All rights reserved.